How close are we to distinguishing between black holes and neutron stars from stellar collapse using gravitational waves?

Are we truly pushing the limits of our knowledge about the universe? What was once considered science fiction has become a crucial focus in astrophysical research: understanding stellar collapse through gravitational wave frequencies. The mysterious realms of black holes and neutron stars, subjects of deep scientific interest, continue to challenge our understanding. Recent advances in gravitational wave distinguishing technologies have brought us closer to differentiating between black holes and neutron stars than ever before. Based on the latest studies, we will scrutinize how these waves are advancing our knowledge of stellar collapse and scrutinize how close we are to uncovering the ultimate fate of collapsing stars.
The Realm of Black Holes and Neutron Stars
Neutron stars and black holes represent some of the most exotic entities in the universe. A neutron star, theoretically the remnant core of a supernova, packs the mass of our Sun into a sphere with a radius of about ten kilometers, rendering its matter extraordinarily dense. Black holes, on the other hand, are regions where gravity has become so intense that not even light can escape, resulting in a singularity surrounded by an event horizon. The fundamental difference between these objects hinges on their masses and the resulting end states of stellar evolution.
Edited video with music of the 4k neutron star merger simulation. Credit: NASA/AEI/ZIB/M. Koppitz and L. Rezzolla
Neutron stars are often observed through their interaction with companion stars in binary systems, where they can exhibit phenomena such as intense X-ray emissions. The Rossi X-ray Timing Explorer‘s discovery of kilohertz oscillations in neutron stars has provided a novel means to probe their properties. These oscillations reveal the rapid intensity variations in X-ray emissions, suggesting that these stars possess unique characteristics that are crucial for understanding their structure and behavior.
In contrast, black holes, which can vary widely in mass, from stellar-mass black holes to supermassive ones in galactic centers, are typically detected via their interactions with nearby matter. When black holes accrete material from a companion star, the matter forms an accretion disk that emits X-rays. The detection of gravitational waves from the mergers of black holes has opened a new observational window, enabling scientists to infer the properties of these otherwise invisible objects.
The Role of Gravitational Waves
Gravitational waves, ripples in spacetime predicted by Einstein’s theory of general relativity, have revolutionized our ability to study stellar remnants. These waves are produced by accelerating masses, such as during the merger of two black holes or neutron stars. The Laser Interferometer Gravitational-Wave Observatory (LIGO) and its international partners, Virgo and KAGRA, have enabled us to “hear” these cosmic events, providing unprecedented understanding into the dynamics of stellar collapse.
One of the significant challenges in this field is distinguishing between the gravitational wave signals produced by different astrophysical processes. For instance, the detection of gravitational waves from the merger of neutron stars, like in the event GW 170817, has provided valuable data on neutron star properties and has even been linked to observations of electromagnetic radiation from the same event. However, identifying the gravitational wave signature of a core-collapse supernova—where a star’s core rapidly compresses to form a black hole or neutron star—has proven more elusive.
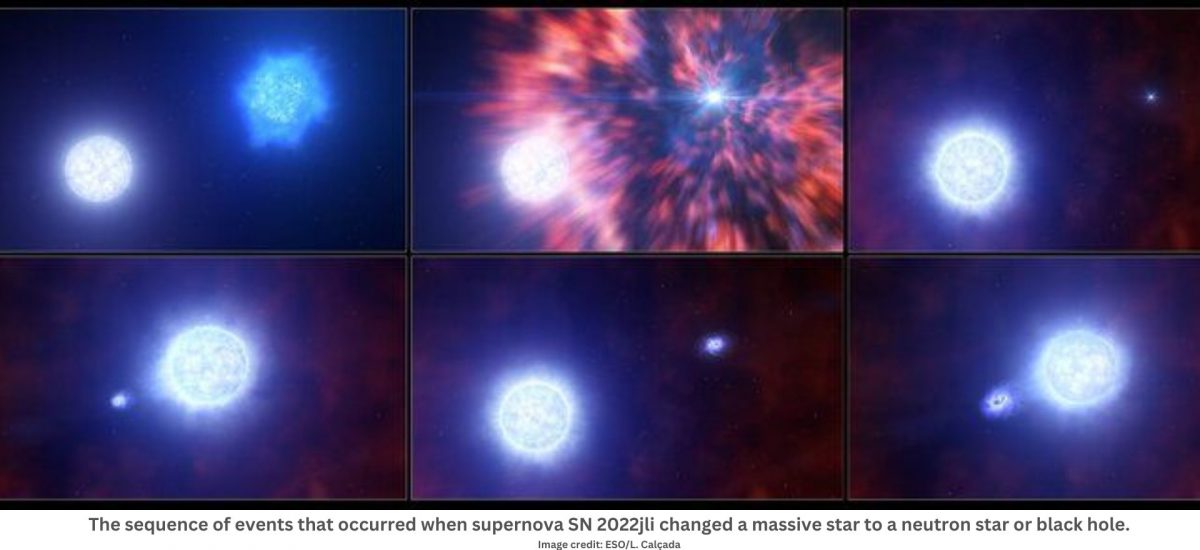
Research has suggested that with advancements in gravitational wave detectors, we might be able to “hear” core-collapse supernovae at distances up to 65 million light-years, potentially offering clues about the final state of the stellar core. This capability would mark a huge leap in our observational reach, but as of now, such detections have not yet been realized.
Advancements in Observational Technology
The current generation of gravitational wave detectors has substantially enhanced our ability to probe cosmic events. The LIGO-Virgo-Kagra (LVK) collaboration has pushed the boundaries of what is detectable, aiming to observe gravitational waves from a variety of sources. Despite these advancements, detecting gravitational waves from core-collapse supernovae remains a challenge due to the expected low frequency of these events and their comparatively weak signals.

Researchers have estimated that under optimal conditions, we could potentially detect up to one core-collapse supernova event per year, though more conservatively, the rate might be just a few per decade. This estimation underscores both the potential and limitations of current technology in capturing these elusive signals. The challenge, according to the scientists, lies in distinguishing these signals from the myriad other cosmic sources that gravitational wave detectors measure.
However, the ability to detect and analyze gravitational waves from core-collapse supernovae would significantly enhance our understanding of the final stages of stellar evolution. These observations could help determine whether a collapsing star ends up as a black hole or neutron star. For instance, the mass and rotational characteristics of the remnants can influence the type of compact object that forms. In particular, rapidly rotating black holes surrounded by dense matter could produce distinctive gravitational wave signals that offer clues about their angular momentum and the surrounding torus of matter.
The importance of this research cannot be overstated. By deciphering the gravitational wave signatures of supernovae, scientists could gain insights into the conditions leading to the formation of black holes versus neutron stars. This knowledge has significant implications for our understanding of stellar physics and the lifecycle of massive stars.
Future Challenges
Despite the progress made, several challenges remain in our quest to understand stellar collapse through gravitational waves. The sensitivity of detectors needs to improve further to distinguish the faint signals from core-collapse supernovae amidst the noise of other cosmic events. Moreover, the interpretation of gravitational wave data requires sophisticated models that account for various factors, such as the rotational state of the stellar remnant and the interaction of the resulting matter with its environment.
Future advancements in detector technology and data analysis techniques hold the promise of addressing these challenges. The development of next-generation gravitational wave observatories, such as the proposed space-based LISA (Laser Interferometer Space Antenna) mission, could expand our observational capabilities even further. These progresses would eventually enable scientists to probe deeper into the cosmos and refine our understanding of the final stages of stellar evolution.
What’s crucial in exploring black holes and neutron stars through gravitational wave frequencies is that it represents an ever-evolving frontier in astrophysical research, where combining observations from X-ray astronomy and gravitational wave astronomy offers a comprehensive view of these mysterious objects.